Quantum processing units (QPUs) are the quantum equivalent of microcontrollers (MCUs) in electronic equipment. QPUs and the quantum computers that use them are evolving and heading toward large-scale computing. This FAQ looks at current quantum computing architectures and how they are evolving and reviews currently available QPUs and efforts to develop QPUs based on superconductivity and cryo CMOS and to scale up the production of qubits and QPUs.
Advanced quantum computers have less than 100 qubits and can use sophisticated cabling to connect from the cryogenic environment to a room-temperature controller. The complexity increases linearly with the number of qubits and rapidly becomes unwieldy, making it difficult to maintain the qubits at the required millikelvin (mk) temperatures. The needed analog control signals are subject to interference from noise and can experience transmission delays. Two of the possible solutions include the use of cryo-CMOS or superconducting electronics that will enable the control circuitry to be in the cryostat along with the qubits.
Cryo CMOS
Multiple efforts are underway to develop cryo CMOS technology for qubit control and other applications. In addition to the numerous challenges related to modeling and designing CMOS that can operate at mK temperatures and GHz frequencies, there’s the important issue of power consumption and heat generation. Advanced cryo refrigerators are limited to a few W at 4 k and under 1 W at sub-K levels. That limits the power consumption of a QPU to a few mW or less per qubit. In one case, a prototype QPU has been developed that dissipates only 18 nW per cell and is expected to be scalable to at least a few thousand qubits with existing dilution refrigerator technology.
Cryo CMOS is expected to be an important technology for other applications as well as quantum computers. Sensors and other circuitry on satellites could benefit from cryo CMOS. So could amplifiers, sensors, and other devices in high energy physics laboratories, radio astronomy, and a range of medical and chemical instruments like nuclear magnetic resonance (NMR) systems (Figure 1).
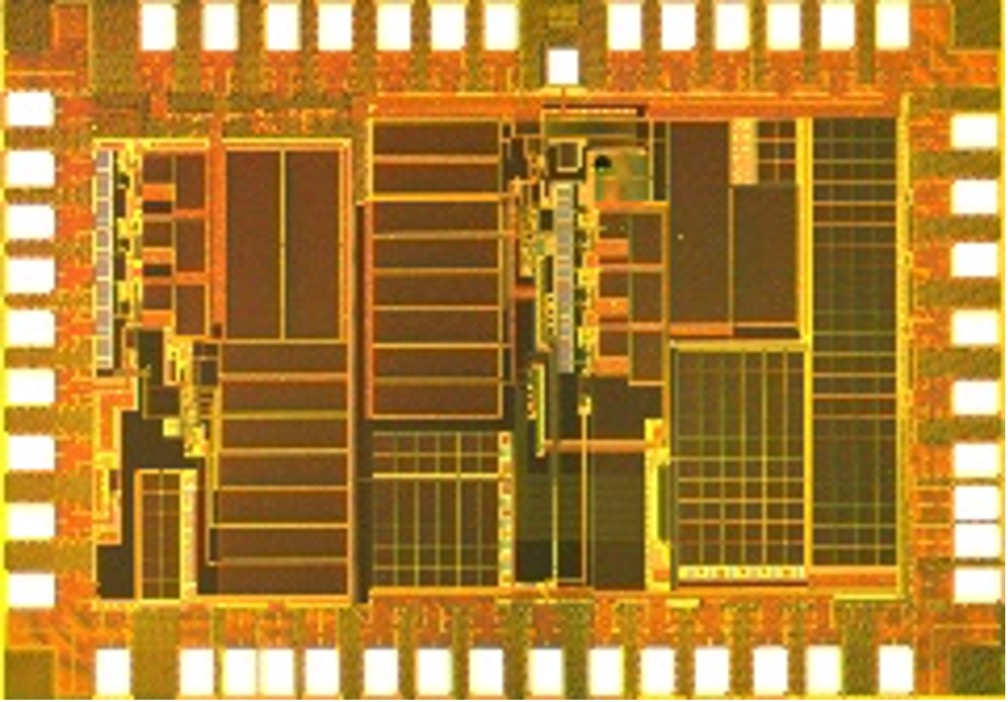
Superconductivity
A device using a superconducting electronics technology called single-flux quantum logic has been developed for qubit control. The device encodes data as a series of single magnetic fluctuations and can operate at up to 40 GHz at 20 mK. It’s compatible with spin qubits as well as superconducting qubit technology. Since the chip operates at about the same temperature as the qubits, there is less heat generated that could degrade qubit operation.
The superconducting controller uses digital control signals that are resistant to corruption from noise. The device also includes a demultiplexer to distribute a single control signal to multiple qubits, reducing the required number of control lines and lessening the challenges when scaling to higher numbers of qubits.
Making qubits on 300 mm wafers
Scaling quantum computers also requires the ability to produce more qubits for a lower cost. Recently, qubits have been fabricated on 300 mm wafers using a process flow like that used for standard CMOS. In these qubits, information is encoded in the spin of individual electrons. These silicon spin qubits are up to 1 million times smaller than other types of qubits and measure about 50 nm square, enabling more efficient scaling and using a series of quantum dot gates for computing. Figure 2 is a schematic representation of an electron under 12 quantum dot gates.
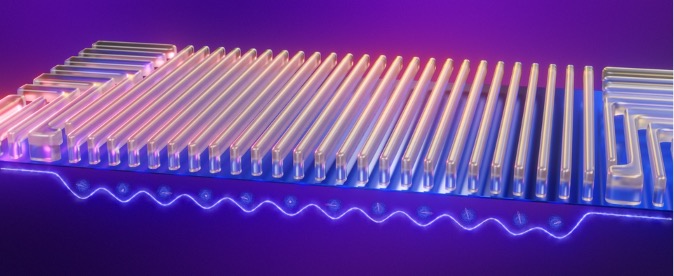
Summary
QPUs are important components in quantum computers and provide the control and interface functions needed for the qubits to be effectively used. Advancements in QPU technology, like the development of superconducting electronics or cryo CMOS, will be needed to enable future scaling of quantum computers to thousands of qubits.
References
Chip Charts Course for Quantum-Computer Scaling, SEEQC
New Chip to Advance Silicon Spin Qubit Research for Quantum Computing, Intel
New spin control method brings billion-qubit quantum chips closer, UNSW Sidney
Quantum Integrated Circuits, TUDelft
Reducing charge noise in quantum dots by using thin silicon quantum wells, Nature Communications