Lidar may become unnecessary for future autonomous vehicles thanks to advances in highly integrated RF chips.
Prajakta Desai, Texas Instruments
Over the years, the application of radar within the automotive industry has improved both safety and convenience. Radar can work in extreme environmental conditions such as rain, snow, dust and bright sunlight and also provide precise distance and velocity information. Consequently, radar is considered the most appropriate sensing method to meet New Car Assessment Program (NCAP) requirements. (NCAP is a government car safety program that aims to evaluate new automobile designs for performance against various safety threats.)
Radar sensing has become a cost-efficient sensing method for advanced driver assistance system (ADAS) functions and to meet Society for Automotive Engineers vehicle autonomy levels 2+ and even 3+. Radar sensors with higher ranges and resolution are evolving to handle more advanced levels of automated driving. And because radar sensors can now support multiple functions, they help on-board controllers better manage space around the vehicle.
Up to level 3+ autonomy, vision and radar sensing can address the requirements economically. (However, wheel speed sensors as used for ABS and related functions will still be required.) For level 4 and beyond, vision, radar, and lidar might be necessary. Radar sensors, when built with cascaded transceivers (a higher number of virtual channels), offer lidar-like performance (higher angular resolution), but at the right cost point.
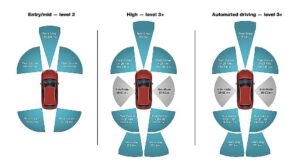
However, the process of safely turning, changing lanes, navigating tight corners all present significant design challenges for advanced vehicle autonomy. In particular, there are technical barriers associated with getting the visibility around corners necessary for designing high-quality ADASs and parking assistance, as well as for broader adoption of autonomous vehicles. Being able to see farther and more clearly with radar leads to improved sensor fusion for safer automated driving and parking. In addition to the performance that radar brings to the table, the key advantage of radar for an ADAS is its ability to operate reliably regardless of weather conditions.
In particular, 77-to-81-GHz millimeter-wave (mmWave) long-range radar sensors from Texas Instruments offer the ability to detect objects in a wide geographical area out to 200 m. Mid-range sensors operate in the range of 100-150 m, while short-range sensors use transceivers, with signal-processing equipment mounted behind the bumper, track an object or person 30-50 m from the vehicle. CMOS technology has enabled a radar front end with a high level of integration.
For short-range radar, the 24-GHz narrow and ultrawide (>500 MHz) bands have been used in legacy automotive sensors. For simple applications such as basic blind-spot detection, you can use industrial, scientific and medical (ISM) band. (Specifically the 2.4 GHz ISM band, because of the readily available and inexpensive wireless ICs working at these frequencies.)
But in most cases, including ultra-short-range radar applications, the need for high resolution dictates use of the ultrawide band. This band is being phased out, however, because of spectrum regulations and standards developed by the European Telecommunications Standards Institute and the U.S. Federal Communications Commission.

The 24-GHz ultrawide band became unavailable as of Jan. 1, 2022, known as the “sunset date,” both Europe and the U.S.; only the narrow ISM band will be available in the long term. This lack of wide bandwidth in the 24-GHz band, coupled with the need for higher performance in emerging radar applications, makes 24 GHz unattractive for new short-range radar implementations.
There is a 76 to 77-GHz band available for vehicular long and mid-range radar applications. This band has the benefit of a high allowed equivalent isotropic radiated power. (The total power needed for an ideal isotropic antenna to produce the same power density as a beam antenna.) The power level is sufficient to enable front long-range radar applications such as adaptive cruise control. The 77 to 81-GHz short-range radar band has recently gained significant traction for uses in industry.
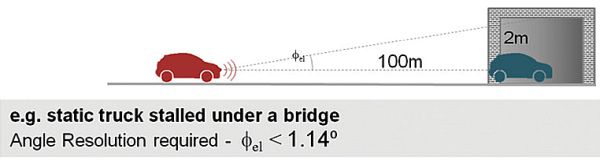
One key benefit of 77 GHz radar is its wide sweep bandwidth, 4 GHz. The range resolution of a radar sensor signifies the minimum dimension to which it can distinguish two closely spaced objects, whereas the range accuracy represents the accuracy in measuring the distance to a single object. Range resolution and accuracy are inversely proportional to the sweep bandwidth. More specifically, with FMCW radar used in vehicles, the frequency change over a given time limit determines the resolution. Thus, due to its ability to accommodate a faster frequency shift, a 77-GHz radar sensor can realize 20 times better performance in range resolution and accuracy compared to 24-GHz radar. The achievable range resolution is 4 cm (versus 75 cm for 24-GHz radar). Moving forward, most automotive radar sensors will likely shift to the 77-GHz band.
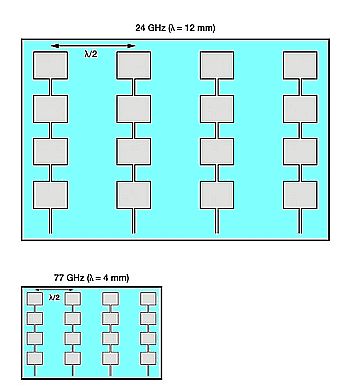
Smaller sensor size is another advantage of higher radar frequencies. For a chosen antenna field of view and gain, the area of the antenna array can be approximately three times smaller than that for 24 GHz. This size reduction is particularly useful in automotive applications where sensors must sit in cramped quarters behind the bumper, in doors and trunks for proximity applications, and inside the cabin for occupant sensing and similar tasks.
As good as lidar
In current ADASs, primary safety measures such as automatic emergency breaking (AEB), autonomous emergency steering (AES), automatic cruise control (ACC) and forward collision warning (FCW) are considered basic features and are mandated by regional NCAP regulations.
As technology progresses, ADASs incorporate more crash avoidance and safety measures. These safety measures depend on the sensors in the underlying systems. Original equipment manufacturers (OEMs) and Tier-1 suppliers pay close attention to the sensor suite for these functions, specifically ensuring that sensors meet the stringent requirements of NCAP tests. And traditionally, the sensor technology used for pedestrian avoidance, lane-change warnings, auto-braking solutions and adaptive cruise control applications has been lidar.
Automotive lidar sensors use light reflected from targets to gauge distance, velocity, and perhaps other features. Lidar sensors can detect objects nearly 200 m in front of the vehicle. High cost is a primary concern when using lidar, however. Fortunately, the combination of vision and radar sensing can cost-efficiently address requirements to autonomy level 3+; but it may take these methods combined with lidar to handle level 4 and beyond.
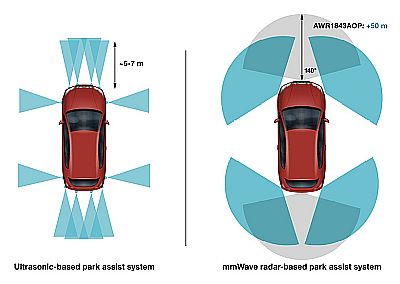
Consider a sensor configuration in which multiple TI AWR2243 sensors are cascaded together. This assembly operates synchronously as one radar unit, with many receive and transmit channels. The result is significantly enhanced angular resolution as well as better radar range. Cascaded together, mmWave sensors can reach an extended range of up to 400 m using integrated phase shifters to create beamforming. Radar sensors built with cascaded transceivers (effectively providing a higher number of virtual channels) offer lidar-like performance (higher angular resolution) at a lower cost.
One place where cascaded radar sensors excel is in identifying static objects with a high resolution. This contrasts with the typical mmWave sensor which is limited in IDing objects standing still.
New applications
To get a 360° surround view of the vehicle environment, auto manufacturers must integrate sensors into small spaces such as door handles or B-pillars. The small form factor of TI’s AWR1843AOP antenna-on-package (AOP) sensor facilitates this process. TI’s AOP mmWave radar sensor integrates the antenna, radar transceiver, digital signal processor, microcontroller and interface peripherals onto one chip. So there is no need for a high-frequency PCB substrate. And the fact the antenna is built-in eliminates antenna development work that speeds time to market.
Today, surround-view cameras and ultrasonic sensors enable parking assistance. But drivers still must make judgments and maneuver based on sensor feedback. To enable parking that is completely automated, the sensor should be capable of detecting other cars, curbs or pedestrians from 3 cm to more than 40 m away in a wide field of view, despite weather conditions. In that regard, mmWave sensors can detect smaller nearby (<25 cm) objects (such as a metal rod protruding from the ground) that other sensing methods might miss, all while functioning under a variety of weather and lighting conditions. Radar sensors also improve vehicle aesthetics because they operate without the need of bumper holes.
Radar sensors can also detect what goes inside the passenger compartment. Driver monitoring systems, seat-belt reminders and airbag deployment are all candidates for radar sensing. Heavy objects laid on a seat need no longer trigger seat-belt reminders. Airbags could deploy at different speeds to accommodate small children. Absent-minded drivers could receive reminders that infants or children are still inside vehicles because radar detects lifeforms more accurately than any other sensor technology.
Radar sensors can also detect drowsy drivers by estimating heartbeat and breathing rates. In fact, a single sensor can monitor vital signs for all occupants and generate alerts about their medical issues.
Also, radar sensors can detect gestures such as the swipe of a hand or twirl of a finger so drivers needn’t take their eyes off the road to fumble for pushbuttons or knobs. Ditto for movie-watching passengers in second row who would no longer need to extend their hands toward a screen to touch a knob.